The world was a very different place during the height of last ice age-known as the Last Glacial Maximum (LGM)-with very different climates, landscapes, and floral and faunal distributions compared to our current epoch, the Holocene. The exact chronology of this period varies according to the source but it is generally considered to be the point during the Last Glacial Period when the continental ice sheets reached their maximum extent and is believed to have lasted a few thousand years with the midpoint being 21 or 20 thousand years ago.
While there is much disagreement about the exact amount, temperatures are generally believed to have been about 4.5 degrees colder globally1. However, anomalies were significantly larger near the enormous ice sheets. The LGM is sometimes thought to serve as a reverse analog to future warming because Earth’s orbital forcing was comparable to today while temperatures and CO2 were far lower2, making it of great interest to scientists. Despite all this interest and research, much remains unknown about the period.
If you’ve read anything related to the LGM whether it be the archeology, paleontology, or paleoclimatology of the time period, you’re most likely familiar with the idea that the world then was not only much colder than at present, but much drier as well. The idea that cold is tightly linked to dryness and warmth with wetness is so deeply ingrained in scientific thought that for the last few decades, it has been practical orthodoxy that the LGM was more arid almost everywhere on Earth3. But is it really true?
A number of excellent and highly compelling papers have provided a different perspective, arguing that the LGM was not nearly as dry as is often claimed and that the traditional signs pointing to aridity may create a false picture, with major implications for glacial periods as a whole too. I am personally grateful for these papers as they finally made Late Quaternary ecology and climate make sense for me.
In this post, I will summarize their work and explain why we need to reassess long-held beliefs about LGM aridity and provide a more nuanced view of Quaternary climate and ecology. A more widespread understanding of these realities will benefit palaeoecological research.
Defining “drier”
Before we explain why the LGM was not as dry as commonly thought, it is important to first define what exactly is meant by being “drier” in the first place. Does being drier mean there is less precipitation (rain, snow) in a given place or time compared to another place or time? If so, you can make the case that the period was drier, since precipitation was indeed lower over the vast majority of the world than it is now3.
However, the problem is that when you apply that same rigid standard to modern day geographical locations, it would suggest that Anchorage in Alaska (417 mm of annual precipitation) is drier than Sacramento in California (460mm) or that Stockholm, Sweden (550mm) is drier than Barcelona, Spain (590mm). Most people will instinctively understand that is not the case simply on the basis of the greener landscapes in and around the northern cities compared to the more southerly two.
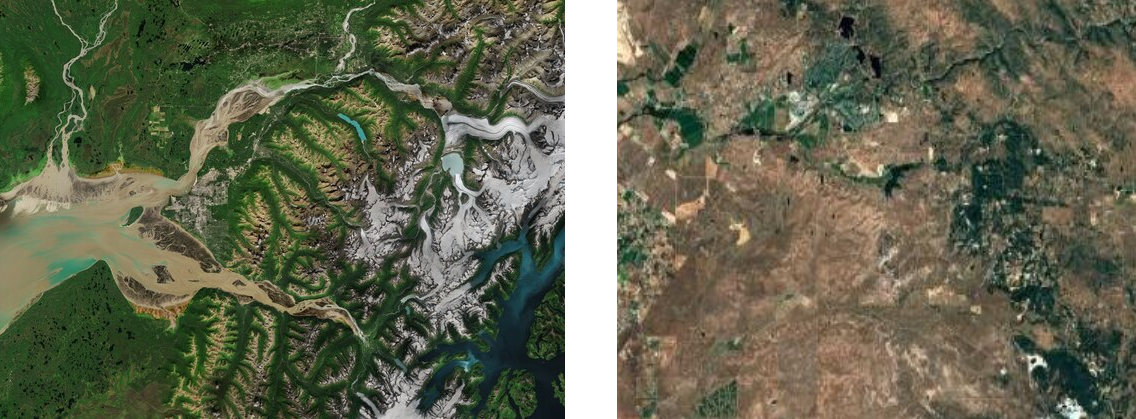
Temperature matters a lot, and even a few degrees difference in temperature-especially in warmer months-can result in substantially different evaporation rates. What we really mean when we think of a place as “drier” is that it is climatically more desert-like, since in deserts the evaporation rate exceeds precipitation. Therefore, cities like Sacramento and Barcelona, due to being much hotter and sunnier than Anchorage and Stockholm, lose much more of the water that falls as precipitation to evaporation than the latter do, causing them to be drier with fewer lakes and less greenery even if precipitation is the same or higher.
That is why the degree of wetness or dryness should be thought of as a balance. Metrics that measure moisture balance like precipitation minus evaporation (P-E) or the precipitation to potential evapotranspiration ratio (P/PET) are more helpful than precipitation alone in determining how dry or wet a place is3.
Is a colder world more arid?
We see why we cannot directly compare the wetness of two places with vastly different temperature ranges, but the same issue arises when comparing the climate of a given place over time i.e. during a warm vs. a cold phase. Yes, precipitation might have been lower during ice ages, but it was also colder and hence there was less evaporation. The question, therefore, is whether temperature dropped in sync with precipitation or if one fell more than the other.
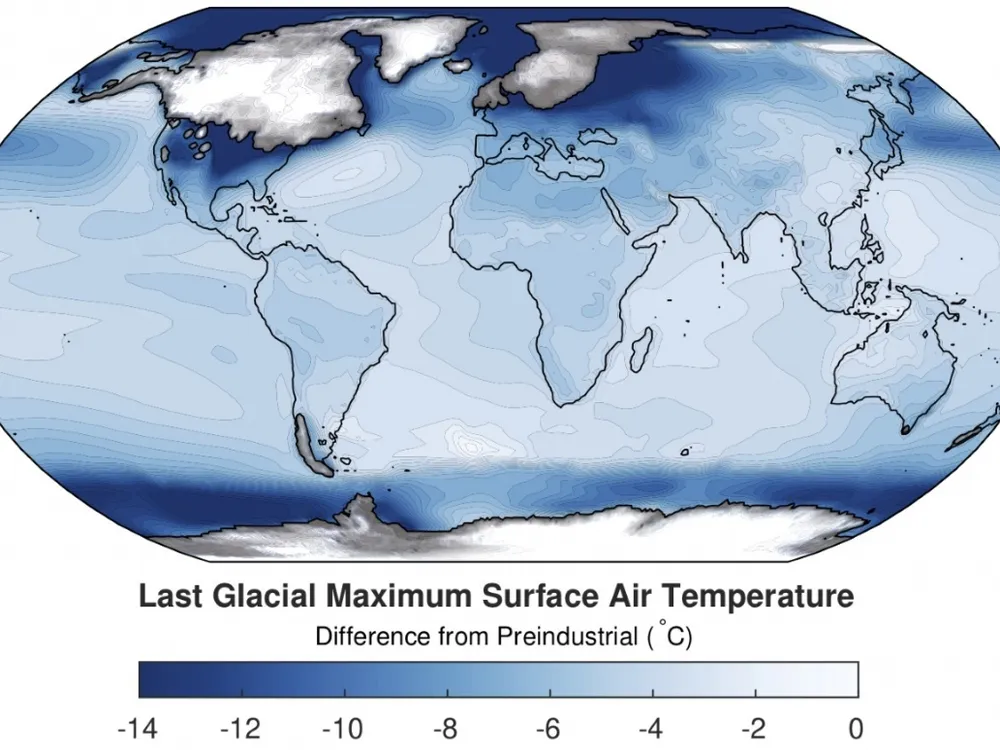
When we use paleoclimate models to reconstruct the LGM climate and then calculate moisture balance, we find that almost the entire world becomes colder but moisture balance responds regionally3 4. Due to thermodynamics, the tropics become drier and the subtropics wetter on average. The massive ice sheets have an effect too, redirecting moisture channels so that the southwestern US and parts of the Mediterranean become wetter while blocking moisture from reaching Siberia and Alaska, making them drier. The exposure of vast continental shelves due to the drop in sea level also results in greater aridity in some places like Indonesia since the distance to sea increases greatly.
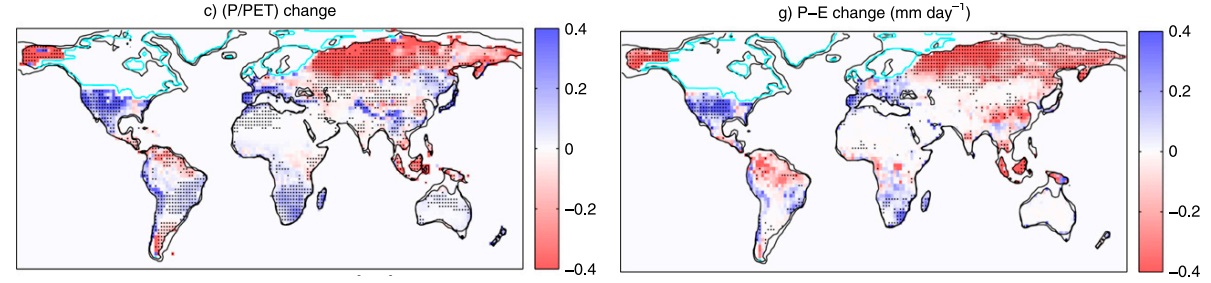
All in all, models suggest some places were drier, others wetter, and the rest not much different. Proxy data such as lake levels seem to suggest that lakes and rivers in many areas were just as full or fuller during the LGM3 4. Therefore, to say that aridity was a consistent pattern of the LGM is quite misleading. Moreover, we know that glaciers need not only prolonged cold but also moisture to grow larger. If greater aridity was so intrinsically linked to cold as many believe, it raises the question of how exactly the ice sheets in North America and Europe ever managed to grow as massive as they did.
I have heard some fellow paleo-enthusiasts claim that ice ages were drier because the massive ice sheets locked up enormous amounts of moisture, but this rationale does not stand upon further inspection. Precipitation ultimately has its roots in the evaporation of ocean water, so the main way this would impact hydrology is by shrinking ocean surface area through lowered sea levels. However, while some areas were more arid as a result of the coastline extending several hundred km further out than today, continental shelf exposure was less than 200 km for most of the world. This meager increase in distance from the sea is certainly not enough to cause alleged widespread aridity.
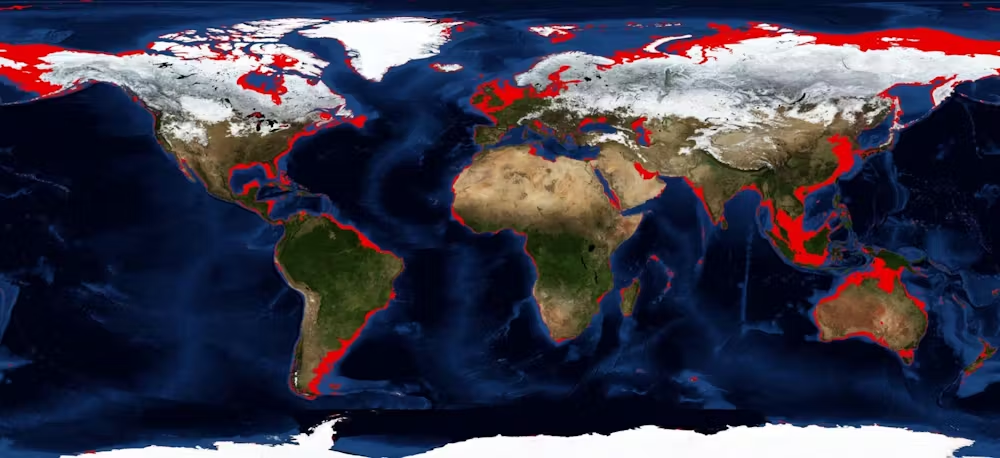
Pollen-based climate reconstructions are unreliable
So we have established the importance of viewing the degree of wetness or dryness as a balance, we can move on to the next issue: pollen reconstructions. Many scientists are aware that precipitation alone is not a good enough measure for how arid or humid a place is, so they turn instead to pollen data. We know from pollen data that drier biomes like grassland and desert expanded at the expense of forest during cold periods like the LGM, and this served as proof for scientists for a long time that ice ages were a lot drier than today5 6. This conflicts with the LGM paleoclimate models and proxy data from lake levels. What explains this?
First, let us discuss how this data on pollen is acquired and analyzed. Scientists take samples of sediments containing pollen. The sediments are dated to different geologic times and the contents are analyzed. Scientists compare the ratio of pollen types to determine what percent of plants were composed of various types of grass, shrubs, coniferous trees, or deciduous trees at any given location at any given time-a sort of environmental snapshot. Based on that composition, they determine to the best of their ability what the climate was like at the time.
The problem is that one cannot reliably compare the climate across time periods like that given the huge variations in CO2 concentrations during the Quaternary. Although CO2 concentration is not considered a climatic metric in the strict sense the way temperature, precipitation, wind, and cloud cover are, it affects both the climate and environment in dramatic ways. The pre-industrial CO2 concentration on earth was about 280 ppm. The concentration of CO2 during the LGM was about 185 ppm, and remained fairly low during the last 70k years in general-mostly between 220 and 190ppm. Concentrations under 200 ppm are particularly challenging for plant survival7. This had a huge effect on the plant composition of glacial environments
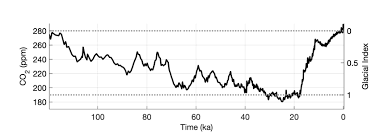
Plants have pores in their leaves called stomata which they use to exchange gas with the atmosphere. This is how they acquire CO2, which is vital for all plants. Under low CO2 conditions, plants must increase the number of stomata so that they capture enough CO2 to fulfill their metabolic needs7. Increasing their stomatal conductance increases water loss to the atmosphere, also known as transpiration.

The critical point is that this does not affect all plants equally: C4 plants require less CO2 than C3 plants while herbaceous (non-woody) ones like grasses and forbs require less CO2 than woody plants like trees and shrubs. Grasses and forbs have an advantage since they do not need to increase stomatal conductance as much, which is why during the LGM we see a preponderance of open/grassy habitats where forests exist today5 6
Now, with high enough moisture balance, forests can still survive under low CO2. After all, we know that rainforests did not die out. But it takes a climate where rainfall/fog is so substantial that plants can easily replenish the water that is lost via increased stomatal conductance. Further, moist tropical vegetation seems to be fairly resilient to changes in CO2 8, which may explain why rainforests continued to thrive in the LGM world. In places which were borderline in terms of moisture balance, a shift towards more open biomes was almost inevitable when CO2 fell too low. Forests would have converted to either forest-steppes or open woodlands, while dry grasslands could turn into deserts.
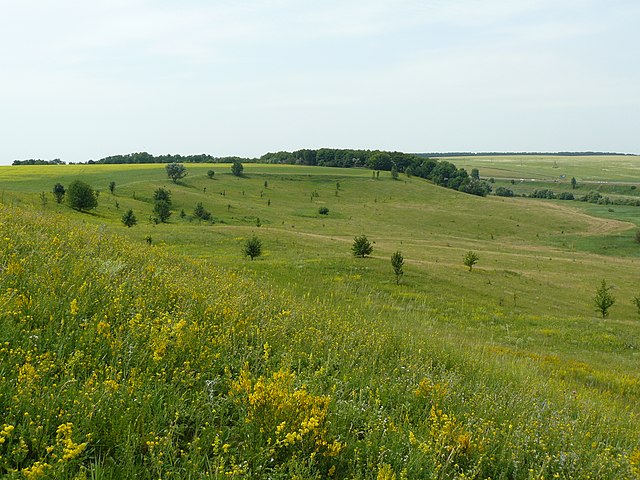
Since CO2 changes have strongly paced temperature changes, with lower CO2 during cold periods, it was hard to tell what was responsible for the changes in vegetation. It was perfectly natural to assume that the cold always resulted in increased aridity and hence more open vegetation, but we now know this is not necessarily the case.
Dustiness
Another deceptive feature of cold periods which causes them to appear drier than they actually were is high dustiness. Significant regions of loess (deposits of wind-blown dust)9 are associated with stadials (colder periods within glacials), while the same periods featured a much greater flow of dust into Antarctica and Greenland compared to the Holocene, as the graph of ice core data below indicates:
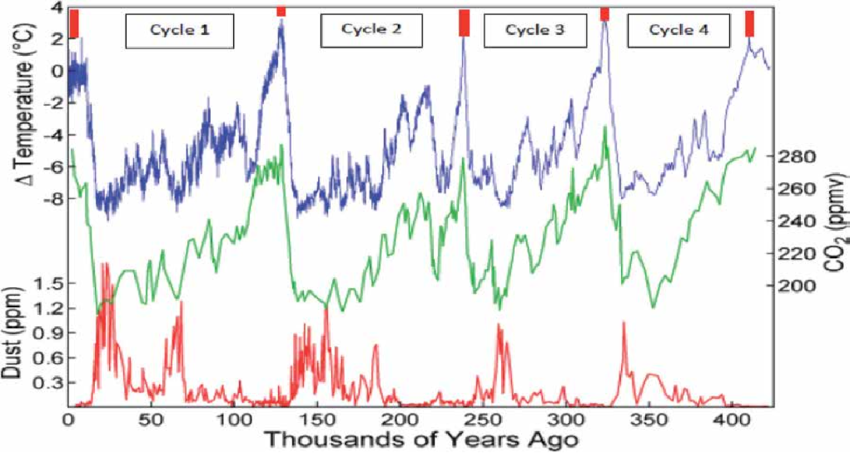
A dustier atmosphere was taken to be yet another sign of aridity because it suggests that there was a greater amount of desert and bare soil at the time9. However, we already know that lower CO2 concentrations can result in desert and semi-desert expanding even with no change in aridity. We also know that winds were much stronger during very cold phases because the temperature gradient between the high and low latitudes was much sharper, resulting in much stronger winds which carried more dust along vast distances4. Therefore, a dusty atmosphere and greater dust deposition are not necessarily proof of a drier climate.
Caveats for other stadials
While this post primarily concerns the LGM, the last glaciation (115-11.7 thousand years ago) as well as previous glaciations featured large periods of fairly low CO2, and plant growth-especially woody- was likewise suppressed compared to the present. Therefore, aridity would appear exaggerated for cold, low CO2 periods in general. This is an important point to consider when analyzing Quaternary environmental changes.
However, it is worth noting that not all cold periods were entirely comparable to the LGM in every way. There were some like the Younger Dryas and Heinrich stadials which were quite cold and do show some compelling evidence of having truly been drier in some areas10 11. The Younger Dryas at the end of the Pleistocene around 12.9-11.7 thousand years ago is notable as it was a unique stadial wherein CO2 was not low (around 240 ppm). Therefore, proper climate variables like precipitation and temperature rather than CO2 would be responsible for the more open vegetation compared to the warmer periods before and after it.
These stadials differed from the LGM because they were the result of a collapse in the thermohaline circulation as a result of a large influx of freshwater into the North Atlantic, ending transport of warm water and causing sea temperatures to plummet off of western Europe to a greater extent than during the LGM12 13. The Younger Dryas may have also produced El Niño-like conditions over the Pacific, resulting in low sea temperatures there as well11. Cold seas are bad for moisture production, which could explain the relative aridity of these stadials in some places.
Changes in insolation across glacial periods can also have impacts. These temporal differences are important to take into account to give a full picture of Quaternary hydrology.
The mammoth steppe in context
Moving on to the last subject: what can this research about LGM climate, atmosphere, and vegetation tell us about the largest and most famous Pleistocene biome, the mammoth steppe? The mammoth steppe (also known as steppe-tundra) was a biome whose distinctive floral characteristics and animal community-woolly mammoths, woolly rhinos, saiga, caribou, muskoxen, cave lions, and more-have captivated the imaginations of those who are curious about the Pleistocene, especially paleontologists14.
There is a degree of ambiguity as to how the mammoth steppe is defined but it is generally understood to be an ecosystem with no or few modern analogs-a mixture of tundra and steppe-which used to stretch from western Europe to the Yukon before disappearing at the end of the Pleistocene to be replaced by boreal forest and tundra. The reasons for its disappearance are not fully clear.
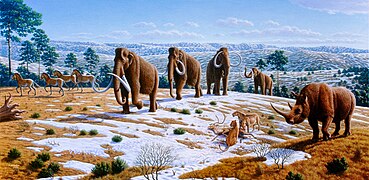
Some scientists argue that it was maintained by its cold and dry climate. R. Dale Guthrie, who has perhaps done the most prolific research on the biome, emphasizes the particular importance of clear skies in producing the aridity necessary to give herbaceous taxa dominance over trees and shrubs14. According to Guthrie’s hypothesis, climate change towards a wetter/cloudier climate is what caused the mammoth steppe to disappear along with its denizens.
Other scientists, such as Sergey Zimov, believe that animals were the critical force in maintaining the mammoth steppe by disrupting snow cover and trampling and eating shrubs and trees15. He believes that the extinction of the animals is what caused the biome to be replaced by boreal forest and shrubby tundra. Are either of these explanations fully sufficient?
Starting with the climate argument, not all of the glacial era steppe-tundra or adjacent environments, like boreal forest-steppe, were especially dry, at least during the LGM when they were most widespread. All modern Arctic tundras are dominated by shrubs, mosses, and sedges15, yet western portions of Europe appear to have received decent precipitation and were covered with steppe-tundra vegetation as opposed to more modern types of tundra. In warmer parts of Europe closer to the Mediterranean, forest-steppe dominated rather than true forest.
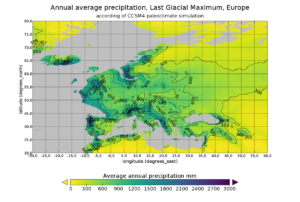
Guthrie argues that clear skies were more critical than precipitation in producing aridity, but realistically, areas receiving 500 mm or more of precipitation (see map above) under a cold climate regime will have plenty of cloudy days anyway. To be fair to Guthrie, most of his research centered on Beringia where glacials were indeed quite dry, but different explanations are needed for wetter parts of the mammoth steppe.
Zimov correctly ascertains that vast areas of the northern latitudes have climates that fall within the range of those present in the mammoth steppe, terming this the “mammoth steppe climatic envelope”17. To explain why these areas no longer support this biome, he has argued that the extinction of the herbivores was the explanation. It sounds plausible, but the lack of herbivores can’t be the whole reason: Zimov has created a park in Siberia and filled it with large herbivores to recreate the mammoth steppe, but results so far have unfortunately not been promising18. Additionally, studies from Africa suggest that abundant large animals cannot shift the distribution of biomes at the continental level19.
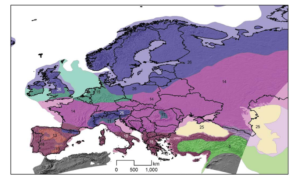
There is still some evidence that suggests large herbivores may have had major impacts on their environments in the past20. Yet, clearly neither climate nor megafauna can fully explain why the mammoth steppe was so widespread or why it eventually disappeared, so what does? That is where CO2 comes in. Because low CO2 in glacials means woody plants struggle to survive, you can have a reasonably high input of moisture while denying woody vegetation the competitive advantage they would normally have.
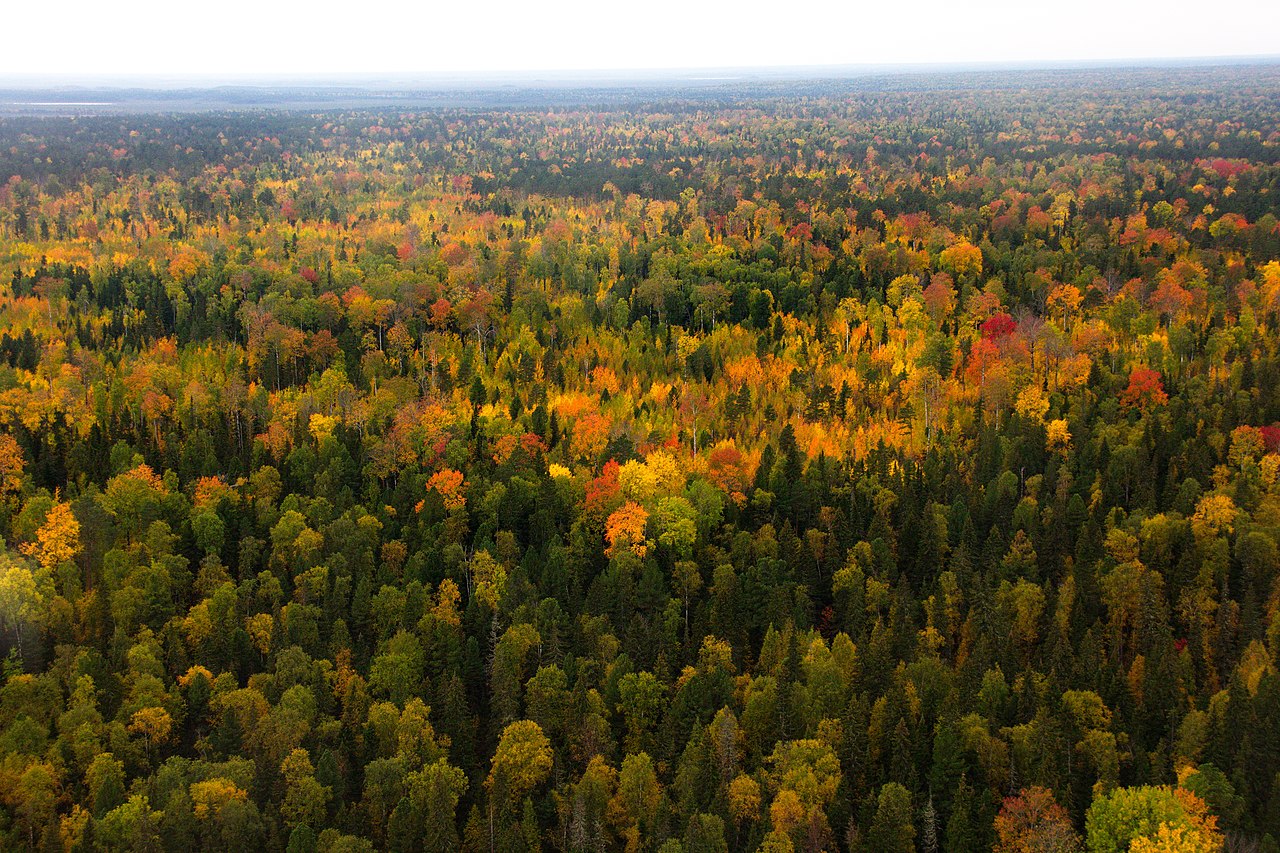
Indeed, paleovegetation models indicate that while a large band of grassland exists across northern Eurasia under an LGM climate with high CO2, it expands massively in an LGM climate with low CO26. A large increase in CO2 during the transition to the Holocene, combined with increased moisture input in certain areas21, is likely the main reason for its disappearance. I do not believe this fully explains why so many mammoth steppe fauna went extinct considering they survived previous interglacials, but it could explain why their habitat shrank so massively.
Conclusion
To sum it up, the Last Glacial Maximum (LGM) was most likely not as dry as we thought despite large regional variations. It is important to be precise in how we define wet or dry in the context of climate, and cold is not necessarily intrinsically linked to dryness. CO2 concentration was a significant force in Quaternary environmental dynamics, and climate alone is not a sufficient way to explain shifts in flora and fauna in a given location. Lastly, the mammoth steppe biome which stretched across Eurasia and part of North America should be viewed partly as the product of a low CO2 atmosphere.
I felt this post was worth making because an appreciation of the role of CO2 is still lacking to a large extent. Over the years, I have come across palaeoecological studies which unfortunately did not factor CO2 into their analyses. The importance of CO2 concentrations in shaping ecosystems has been known by many scientists for a while but has been slow to catch on among other researchers as well as science communicators. Still, awareness of its profound impact does appear to be gaining steam from what I can tell. Hopefully, this trend continues as it will result in a better understanding of natural history.
References
1. Annan, J. D., Hargreaves, J. C., & Mauritsen, T. (2022). A new global surface temperature reconstruction for the Last Glacial Maximum. Climate of the Past, 18(8), 1883–1896. https://doi.org/10.5194/cp-18-1883-2022
2. Lowry, D. P., & Morrill, C. (2018). Is the Last Glacial Maximum a reverse analog for future hydroclimate changes in the Americas? Climate Dynamics, 52(7-8), 4407–4427. https://doi.org/10.1007/s00382-018-4385-y
3. Scheff, J., Seager, R., Liu, H., & Coats, S. (2017). Are Glacials Dry? Consequences for Paleoclimatology and for Greenhouse Warming. Journal of Climate, 30(17), 6593–6609. https://doi.org/10.1175/jcli-d-16-0854.1
4. McGee, D. (2020). Glacial–Interglacial Precipitation Changes. Annual Review of Marine Science, 12(1), 525–557. https://doi.org/10.1146/annurev-marine-010419-010859
5. Prentice, I. C., & Harrison, S. P. (2009). Ecosystem effects of CO2 concentration: evidence from past climates. Climate of the Past, 5(3), 297–307. https://doi.org/10.5194/cp-5-297-2009
6. Woillez, M.-N. ., Kageyama, M., Krinner, G., de Noblet-Ducoudré, N., Viovy, N., & Mancip, M. (2011). Impact of CO2 and climate on the Last Glacial Maximum vegetation: results from the ORCHIDEE/IPSL models. Climate of the Past, 7(2), 557–577. https://doi.org/10.5194/cp-7-557-2011
7. Gerhart, L. M., & Ward, J. K. (2010). Plant responses to low [CO2] of the past. New Phytologist, 188(3), 674–695. https://doi.org/10.1111/j.1469-8137.2010.03441.x
8. Gosling, W. D., Miller, C. S., Shanahan, T. M., Holden, P. B., Overpeck, J. T., & Frank van Langevelde. (2022). A stronger role for long-term moisture change than for CO2in determining tropical woody vegetation change. Science, 376(6593), 653–656. https://doi.org/10.1126/science.abg4618
9. Muhs, D. R. (2013). The geologic records of dust in the Quaternary. Aeolian Research, 9, 3–48. https://doi.org/10.1016/j.aeolia.2012.08.001
10. Obreht, I., Wörmer, L., Brauer, A., Wendt, J., Alfken, S., De Vleeschouwer, D., Elvert, M., & Hinrichs, K.-U. (2020). An annually resolved record of Western European vegetation response to Younger Dryas cooling. Quaternary Science Reviews, 231, 106198. https://doi.org/10.1016/j.quascirev.2020.106198
11. Hong, B., Hong, Y., Uchida, M., Shibata, Y., Cai, C., Peng, H., Zhu, Y., Wang, Y., & Yuan, L. (2014). Abrupt variations of Indian and East Asian summer monsoons during the last deglacial stadial and interstadial. Quaternary Science Reviews, 97, 58–70. https://doi.org/10.1016/j.quascirev.2014.05.006
12. Martin-Garcia, G. (2019). Oceanic Impact on European Climate Changes during the Quaternary. Geosciences, 9(3), 119. https://doi.org/10.3390/geosciences9030119
13. Eynaud, F., de Abreu, L., Voelker, A., Schönfeld, J., Salgueiro, E., Turon, J., Penaud, A., Toucanne, S., Naughton, F., Sánchez Goñi, M. F., Malaizé, B., & Cacho, I. (2009). Position of the Polar Front along the western Iberian margin during key cold episodes of the last 45 ka. Geochemistry, Geophysics, Geosystems, 10(7). https://doi.org/10.1029/2009gc002398
14. Guthrie, R. D. (2001). Origin and causes of the mammoth steppe: a story of cloud cover, woolly mammal tooth pits, buckles, and inside-out Beringia. Quaternary Science Reviews, 20(1–3), 549–574. https://doi.org/10.1016/s0277-3791(00)00099-8
15. Blinnikov, M. S., Gaglioti, B. V., Walker, D. A., Wooller, M. J., & Zazula, G. D. (2011). Pleistocene graminoid-dominated ecosystems in the Arctic. Quaternary Science Reviews, 30(21-22), 2906–2929. https://doi.org/10.1016/j.quascirev.2011.07.002
16. Zimov, S. A., Zimov, N. S., Tikhonov, A. N., & Chapin, F. S. (2012a). Mammoth steppe: a high-productivity phenomenon. Quaternary Science Reviews, 57, 26–45. https://doi.org/10.1016/j.quascirev.2012.10.005
17. Zimov, S. A., Zimov, N. S., Chapin, F. S., Iii, & Zimov, S. A. (2012). The Past and Future of the Mammoth Steppe Ecosystem. Paleontology in Ecology and Conservation. https://doi.org/10.1007/978-3-642-25038-5_10
18. Reinecke, J., Ashastina, K., Kienast, F., Troeva, E., & Wesche, K. (2021). Effects of large herbivore grazing on relics of the presumed mammoth steppe in the extreme climate of NE-Siberia. Scientific Reports, 11(1), 12962. https://doi.org/10.1038/s41598-021-92079-1
19. Pachzelt, A., Forrest, M., Rammig, A., Higgins, S. I., & Hickler, T. (2015). Potential impact of large ungulate grazers on African vegetation, carbon storage and fire regimes. Global Ecology and Biogeography, 24(9), 991–1002. https://doi.org/10.1111/geb.12313
20. Pearce, E. A., Mazier, F., Normand, S., Fyfe, R., Andrieu, V., C. C. Bakels, et al. (2023). Substantial light woodland and open vegetation characterized the temperate forest biome before Homo sapiens. Science Advances, 9(45). https://doi.org/10.1126/sciadv.adi9135
21. Monteath, A. J., Gaglioti, B. V., Edwards, M. E., & Froese, D. (2021). Late Pleistocene shrub expansion preceded megafauna turnover and extinctions in eastern Beringia. Proceedings of the National Academy of Sciences, 118(52). https://doi.org/10.1073/pnas.2107977118